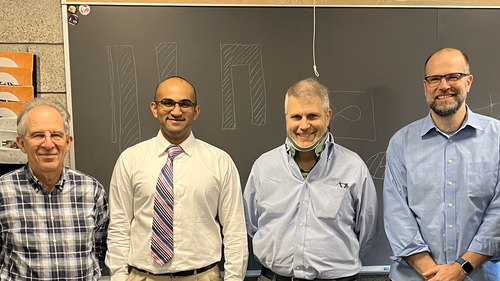
On October 18, 2023, Suhas Ganjam, successfully defended the thesis, “Improving the Coherence of Superconducting Quantum Circuits through Loss Characterization and Design Optimization” (advisor: Robert Schoelkopf).
Ganjam explained, “In order to build a practical quantum computer, we need to improve the ability for quantum bits, or qubits, to retain the information encoded in them, also known as coherence. In the superconducting qubits platform, energy loss is one of the most significant sources of decoherence. However, a comprehensive and quantitative understanding of energy loss mechanisms does not yet exist. It has therefore remained a significant challenge to systematically analyze the various sources of energy loss in superconducting qubits and optimize circuits to maximize performance. My thesis work focused on addressing this issue by developing experimental platforms that use microwave resonators to understand losses in superconducting quantum circuits. With this approach, we can systematically study what limits coherence and optimize materials, fabrication processes, and circuit design to maximize qubit performance. These techniques can be utilized to assist in the development of large-scale quantum processors with lower error rates.”
Ganjam will join Google as a research scientist where he will join the Quantum AI team and help develop a practical quantum computer.
Abstract: Realizing a practical quantum computer with superconducting qubits requires substantially higher gate fidelities, which necessitates further improvements in the coherence of superconducting quantum circuits. Steady improvements have been made over the past two decades, including substantial exploration into energy relaxation mechanisms in superconducting thin films. However, a comprehensive and quantitative understanding of the relative impact of these mechanisms does not yet exist. In this thesis, I utilize a multimode approach to systematically characterize microwave losses in the quantum regime, with the goals of understanding relaxation-limiting loss mechanisms and improving device coherence through materials, process, and circuit design optimization. Using this approach, we measure significant reductions in surface losses by employing a tantalum-based materials platform, and in bulk substrate loss by utilizing high-temperature annealing processes. With this knowledge we predict and experimentally verify the relaxation times of aluminum and tantalum-based transmon qubits. We additionally optimize device geometry to maximize coherence within a coaxial tunnel architecture, and realize on-chip quantum memories with single-photon Ramsey times of 2.0-2.7 ms, limited by their energy relaxation times of 1.0-1.4 ms. This demonstrates an important link between microwave loss characterization and improving coherence in superconducting qubits, and enables a more modular and compact coaxial circuit architecture for bosonic qubits with reproducibly high coherence.