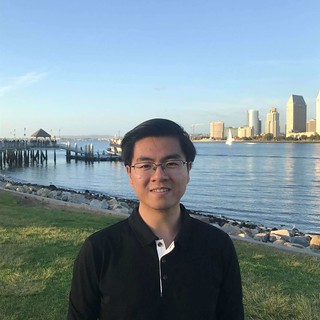
Quantum control of an oscillator with a Kerr-cat qubit
Noise presents a major obstacle to scaling up quantum computers, causing logical errors and preventing the reliable execution of quantum algorithms. Quantum error correction provides a path toward fault-tolerance, but this typically comes at the cost of significant resource overhead. Bosonic codes offer a hardware-efficient strategy for quantum error correction by redundantly encoding quantum information in the large Hilbert space of a harmonic oscillator. These codes have been employed to achieve landmark experimental demonstrations. However, these demonstrations are limited by transmon ancilla errors propagating to the encoded logical qubit during syndrome measurements. The Kerr-cat qubit has been proposed as a novel ancilla for these codes due to its theoretically exponential noise bias, which would enable fault-tolerant error syndrome measurements. However, these measurements require coupling between an oscillator and a Kerr-cat qubit. This coupling has only been demonstrated with a transmon qubit. Can we coherently control an oscillator with a Kerr-cat qubit?
In this thesis, we address this question by experimentally realizing a driven parametric coupling of a Kerr-cat qubit to a high-quality-factor microwave cavity with an experimental setup designed and fabricated in-house. This coupling completes a gate set that enables universal quantum control of the cavity. We measure the decoherence of the cavity in the presence of the Kerr-cat and discover excess dephasing due to heating of the Kerr-cat to excited states. By engineering a novel frequency-selective dissipation to counteract this heating, we are able to eliminate this dephasing, thereby demonstrating a high on-off ratio of control. Our Kerr-cat results pave the way toward fault-tolerant measurements of error syndromes of bosonic codes.
Thesis committee: Michel Devoret (advisor), Robert Schoelkopf, Steven Girvin, Peter Rakich, and Alexander Grimm (external)