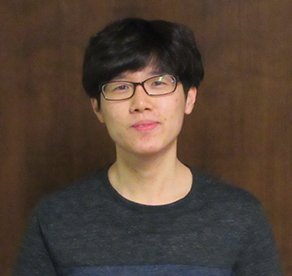
On September 24, 2021, Hyungki (Andrew) Shim successfully defended the thesis “Probing the Upper Limits to Light–Matter Interactions: from Spontaneous Emission to Superresolution”. (Advisor: Owen Miller).
Shim states, “How much light can be radiated from an object of arbitrary size and shape? What materials should one choose for best performance? My thesis maps out what is possible across a wide spectrum of light–matter interactions, addressing such broad questions on the way. One way to search for high-performing structures is to simply sweep over all possible design space via e.g. large-scale optimization tools. However, we can never know for sure if the optimal designs obtained are the best possible—what if some better designs are lurking out there that we happened to miss? To this end, we start from fundamental physical principles and establish upper limits on various optical and nanophotonic quantities of interest. The fundamental limits obtained in this way 1) provide benchmarks against which to compare state-of-the-art devices, 2) suggest pathways for better designs, and 3) conclusively map out the upper bounds that no physical devices will ever be able to surpass.”
On his future plans, “The major theme of my PhD research ,as reflected in my thesis, was to tackle problems in nanophotonics by applying different mathematical and optimization techniques. While I am still exploring my career path after graduation, I wish to leverage my quantitative background to tackle real-world problems. As of today, I am mainly looking for opportunities in data science / machine learning as well as research positions outside academia.”
Thesis Abstract: As newly emerging optical devices and metamaterials inundate the photonics community, there is a growing need to understand the limits to device performance. Are current state-of-the-art designs already near the frontiers of what is possible, or can they be further improved with better technology? Addressing such broad, foundational questions calls for a new paradigm, a novel approach to explore the unimaginably vast, physically allowed design space. In this thesis, we will establish fundamental limits to light–matter interactions in the context of wave propagation in free space via beam shaping, scattering phenomena involving designable structures, and intrinsic properties of optical materials.
First, we derive upper bounds to free-space concentration of light, mapping out the limits to the maximal intensity for any spot size and beam-shaping device. We use “inverse design” to discover metasurfaces operating near these limits, achieving up to 90% of maximum possible intensity. We then establish a sum rule for spontaneous emission, a prototypical near-field response, which relates integrated response over all frequencies to a simple electrostatic constant. Going further, we develop an analytical framework to derive upper bounds to near-field response over any bandwidth of interest and material platform. Finally, we derive fundamental limits to the refractive index of any transparent material. To this end, we use the theory of composites to identify metal-based metamaterials that can exhibit small losses and sizeable increases in refractive index over the current best materials.